Strontium Isotopes (Sr) Geochemistry Overview
The element strontium has four naturally occurring isotopes: 84Sr, 86Sr, 87Sr, and 88Sr. Only 87Sr is radiogenic – produced by the β-decay of 87Rb with a half-life of 48.8 billion years. The most abundant strontium isotope is 88Sr, making up approximately 83% of naturally occurring strontium; followed by 10% 86Sr, 7% 87Sr and <1% 84Sr. In geologic and environmental investigations, the ratio of 87Sr/86Sr is typically used because of their similar abundances – 7% and 10%, respectively.
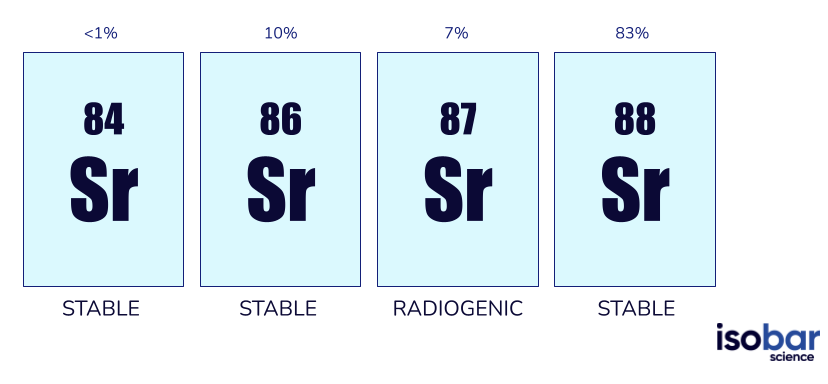
The four strontium isotope species. The ratio 87Sr/86Sr is commonly used in geochemical studies
Strontium is a ubiquitous trace element present in rocks, soil, waters, plants, and animals. The 87Sr/86Sr ratio is a function of the geochemical origin, Rb concentration, and age of the source rock. Because of the high mass of Sr, the ratio is essentially unchanged as the rock weathers and moves through the water cycle and food chain. Thus, the 87Sr/86Sr ratio is extensively used in geochemical fingerprinting, source tracking, contamination prediction, and migration/mobility studies. A systematic change in 87Sr/86Sr ratio of seawater through time has provided a basis for strontium stratigraphy and strontium chronology of marine biogenic carbonates and phosphates.
More information on Sample Types and Selection for strontium analysis.
Strontium Isotopes in Archaeology
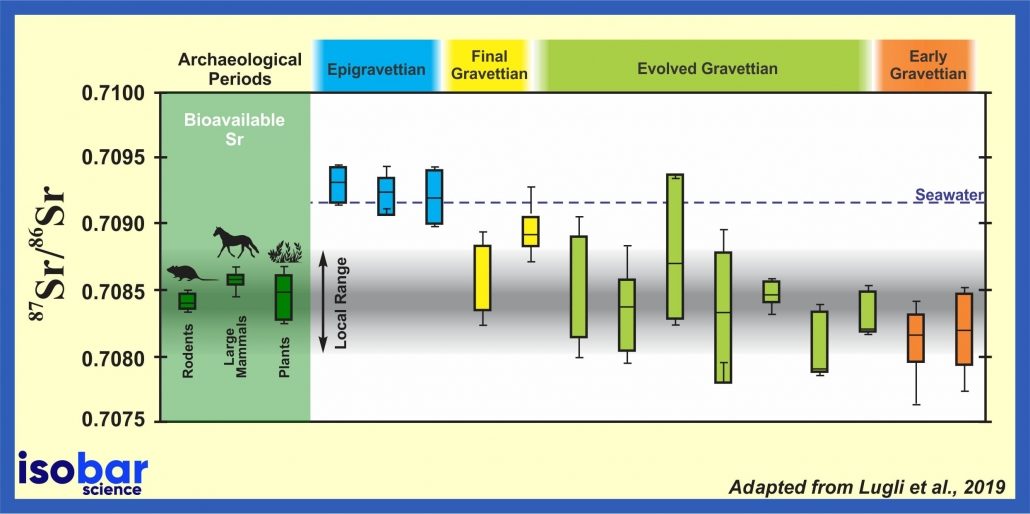
Strontium isotopic ratio (87Sr/86Sr) of human deciduous teeth showing human mobility in southern Italy during the Gravettian and Epigravettian periods (32157-19097 yr cal BP), across the Last Glacial Maximum. Adapted from Lugli et al., 2019.
Each region in the world presents a specific 87Sr/86Sr ratio based on the surrounding geology and water sources. The geology and in turn soils have a specific 87Sr/86Sr signature tied to their origin. Thus, humans, plants, and other animals inherit the Sr isotopic signature of their habitat as Sr is incorporated in their cells along with other elements (i.e. Ca).
As humans and animals move from one habitat to another, they may be exposed to different sources of water and food (plant and animal). Measuring the 87Sr/86Sr ratio in human and animal bone and hair is a powerful tool for tracking mobility and migration. For example, the analysis of 87Sr/86Sr in human teeth can provide information on the location of birth as we are born with both permanent and temporary teeth. Furthermore, 87Sr/86Sr of hair and nails, which are constantly being replenished, can provide evidence of the location later in life prior to death.
Read our posts to learn more:
The use of isotopic analysis on bones: dating, environmental analysis & migration
Tracing the diet of herbivores and omnivores through isotopic analysis to learn more.
Groundwater
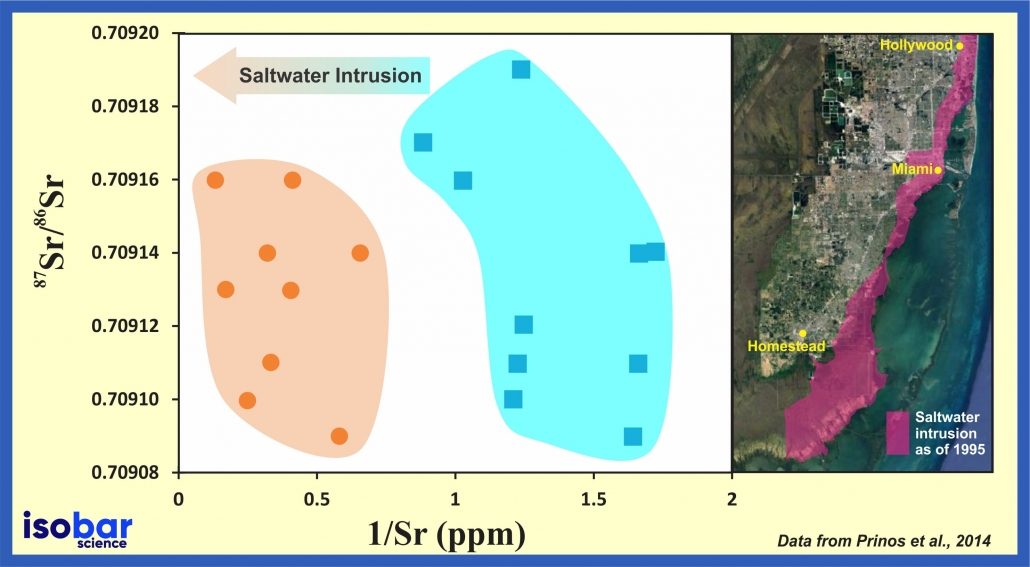
Saltwater intrusion in the Biscayne aquifer as indicated by strontium isotopic ratio (87Sr/86Sr) of water samples collected in Miami-Dade County during 2009. Data from Prinos et al., 2014.
Physical processes such as weathering do not cause fractionation in strontium isotopes. Since the 87Sr/86Sr ratio is not impacted by weathering, it holds the signature of the surrounding rock formations and therefore the type of rocks weathered can also be analysed.
Furthermore, the 87Sr/86Sr ratio of groundwater reflects the isotopic signature of the source water plus the rock formations along its flow path. Using the Sr isotopic ratio along with the Sr concentration in the water is a powerful method to trace groundwater mixing and contamination, as well as saltwater intrusion.
For example, strontium concentration and isotopic values can be used to trace the source and contamination level within groundwater profiles, as demonstrated by Nigro et al. (2017).
Read more about Sr-Nd Isotopes for Weathering Studies.
Geochronology-Stratigraphy
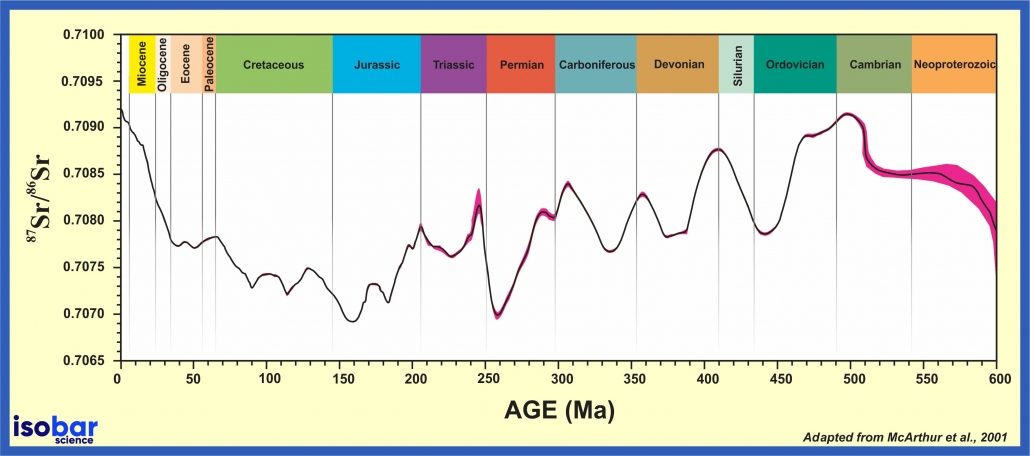
Strontium isotopic ratio ( 87 Sr/ 86 Sr) of seawater during geological time based on analyses of bulk sediment, unaltered brachiopods, belemnites, conodonts and foraminifera samples from various locations around the world. Red area denotes the 95-percent confidence interval. Adapted from McArthur et al., 2001.
Precipitation of marine biogenic carbonates and phosphates involves uptake of strontium from seawater with the 87Sr/86Sr ratio identical to that of the oceanic value at the time of incorporation. This 87Sr/86Sr ratio of the ocean varies through time, creating a predictable seawater curve that can be used for dating calibration. This seawater Sr curve has been developed over the past 850 million years using foraminiferal calcite, belemnite guards, ammonite aragonite, atoll carbonates, and marine cement (McArthur et al. 2012).
By measuring the 87Sr/86Sr ratio in the sample and placing it on the standard seawater Sr-curve the age of the sample (marine minerals) can be determined. This method works best for time periods with long-term unidirectional shifts in the 87Sr/86Sr ratio, such as the Tertiary, but technically can work over the past 600 million years. This method gives a maximum time resolution of 1 million years.
Strontium Isotopes in Forensics
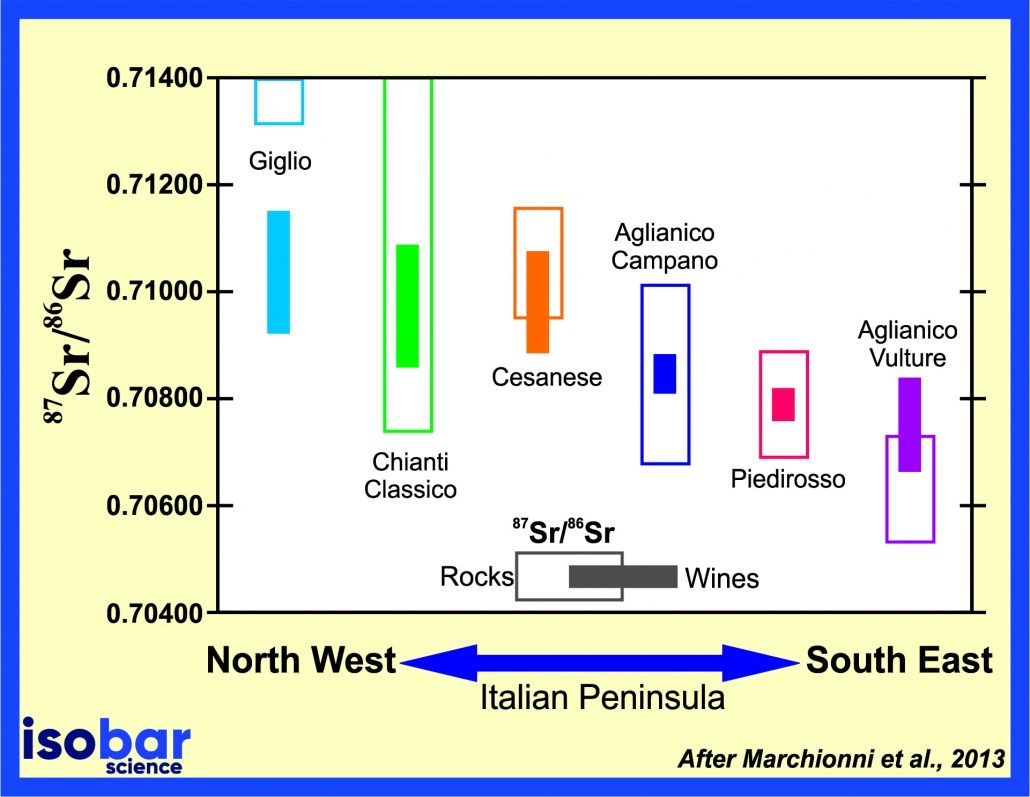
87Sr/86Sr ranges of geological substrates (open boxes) and wines (filled boxes) from the vineyards on the same substrate in different regions of Italy. Redrawn from Marchionni et al., 2013.
The concept of “we are what we eat” is very true when it comes to the isotopic signature of living organisms. The cells of humans, plants, and other animals reflect the isotopic signature of the food and water from their habitats, including the strontium signature of 87Sr/86Sr. This quality has been used successfully in forensic science for the geochemical fingerprinting of narcotics, to study the ethnic background of an individual living in a society, to track the migration pattern of communities, to pinpoint location of death and to trace food adulteration, especially in the wine industry. This technique is similar to that used by archaeological researchers when identifying the migration of people, trade of goods and lifestyle characteristics of previous civilizations.
Read more in our blogs:
Oil Industry
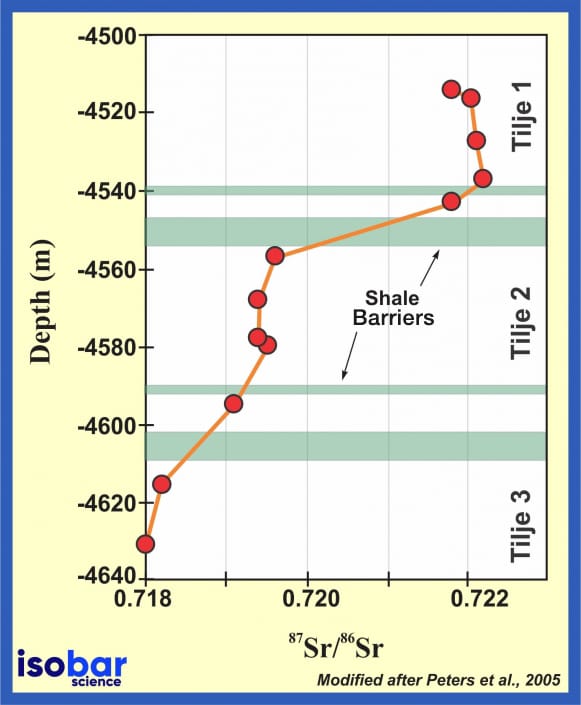
Strontium isotope ratios of residual salts in the oil layer from the lower Jurassic Tilje formation, Norway, show that shale layers compartmentalize oil reservoirs. Some layers are more effective barriers to mixing of petroleum than others. Modified after Peters et al., 2005.
Sr geochemical fingerprinting has a variety of applications in the oil industry. Since different geological formations present specific Sr isotopic signatures, the 87Sr/86Sr ratio can be used to study oil migration and mixing between geological units and their compartmentalization efficiency. The Sr isotopic ratio is also broadly used for production water tracing and isotope stratigraphy.
References
Bentley, R.A., (2006). Strontium isotopes from the earth to the archaeological skeleton: a review. Journal of archaeological method and theory, 13(3), pp.135-187. DOI: 10.1007/s10816-006-9009-x
Capo, R.C., Stewart, B.W. and Chadwick, O.A., (1998). Strontium isotopes as tracers of ecosystem processes: theory and methods. Geoderma, 82(1-3), pp.197-225. DOI: 10.1016/S0016-7061(97)00102-X
Lugli, F., Cipriani, A., Capecchi, G., Ricci, S., Boschin, F., Boscato, P., Iacumin, P., Badino, F., Mannino, M.A., Talamo, S. and Richards, M.P., (2019). Strontium and stable isotope evidence of human mobility strategies across the Last Glacial Maximum in southern Italy. Nature ecology & evolution, 3(6), pp.905-911. DOI: 10.1038/s41559-019-0900-8
Marchionni, S., Braschi, E., Tommasini, S., Bollati, A., Cifelli, F., Mulinacci, N., Mattei, M. and Conticelli, S., (2013). High-precision 87Sr/86Sr analyses in wines and their use as a geological fingerprint for tracing geographic provenance. Journal of agricultural and food chemistry, 61(28), pp.6822-6831. DOI: 10.1021/jf4012592
McArthur, J.M., Howarth, R.J. and Bailey, T.R., (2001). Strontium isotope stratigraphy: LOWESS version 3: best fit to the marine Sr-isotope curve for 0–509 Ma and accompanying look-up table for deriving numerical age. The Journal of Geology, 109(2), pp.155-170. DOI: 10.1086/319243
McArthur, J.M., Howarth, R.J. and Shields, G.A., 2012. Strontium isotope stratigraphy. The geologic time scale, 1, pp.127-144.
Nigro, A., Sappa, G. and Barbieri, M., (2017). Strontium isotope as tracers of groundwater contamination. Procedia Earth and Planetary Science, 17, pp.352-355. DOI: 10.1016/j.proeps.2016.12.089
Peters, K.E., Walters, C.C. and Moldowan, J.M., (2007). The biomarker guide: Volume 1, Biomarkers and isotopes in the environment and human history. Cambridge university press.
Prinos, S.T., Wacker, M.A., Cunningham, K.J. and Fitterman, D.V., (2014). Origins and delineation of saltwater intrusion in the Biscayne aquifer and changes in the distribution of saltwater in Miami-Dade County, Florida (No. 2014-5025). US Geological Survey. DOI: 10.3133/sir20145025
Page last updated: November 2019